Absolute Zero: The Ultimate Temperature Limit
Temperature plays a crucial role in understanding the physical world. It’s a measure of the average kinetic energy of particles in a substance. As we lower the temperature of a system, the motion of its particles slows. This concept leads us to an extraordinary phenomenon: absolute zero—the theoretical lowest temperature in the universe, defined as 0 Kelvin (-273.15°C or -459.67°F). At this point, the motion of particles would theoretically come to a halt.
Understanding Absolute Zero
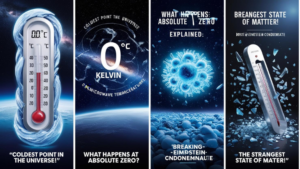
Absolute zero represents the point where a system’s thermal energy is minimized. It’s crucial to note that temperature and particle motion are directly linked. In gases, temperature increases as particles move faster. Conversely, cooling reduces their motion. At absolute zero, the kinetic energy of particles would be minimal, meaning their motion ceases in a classical sense.
However, quantum mechanics challenges this simplistic view. Even at absolute zero, particles exhibit zero-point energy, a quantum phenomenon ensuring that they retain a minimum amount of energy. This prevents the complete cessation of all motion, aligning with Heisenberg’s Uncertainty Principle, which states that the exact position and momentum of a particle cannot be simultaneously determined. This principle implies that particles can never be entirely at rest, even at absolute zero.
Achieving Absolute Zero: The Quest
Reaching absolute zero is a monumental challenge. Scientists have developed advanced methods to cool substances close to this limit. Techniques such as laser cooling and evaporative cooling have been crucial in this quest.
- Laser Cooling: This method uses lasers to slow down particles. When photons from a laser collide with atoms, they transfer momentum, reducing the atoms’ speed and thereby lowering their kinetic energy.
- Evaporative Cooling: This technique involves removing the hottest particles from a system, similar to how evaporation cools water. As the higher-energy particles are removed, the average energy (and thus temperature) of the remaining particles decreases.
Although these techniques bring substances to temperatures near absolute zero, actually reaching it is impossible due to the third law of thermodynamics. This law states that as a system approaches absolute zero, the entropy (or disorder) of the system approaches a constant minimum, requiring an infinite amount of work to remove the final traces of energy.
Applications and Implications
While achieving absolute zero is unattainable, the pursuit has led to groundbreaking discoveries and applications in various fields, particularly in quantum mechanics and condensed matter physics.
1. Bose-Einstein Condensates (BECs)
One of the most fascinating states of matter arises near absolute zero: the Bose-Einstein Condensate. Predicted by Albert Einstein and Satyendra Nath Bose in the 1920s and first observed experimentally in 1995, BECs occur when particles known as bosons occupy the same quantum state. At these ultralow temperatures, individual particles lose their distinct identities and behave as a single quantum entity.
BECs have been instrumental in studying quantum phenomena on a macroscopic scale, leading to advances in understanding superfluidity (where a liquid flows without viscosity) and superconductivity (where certain materials conduct electricity with zero resistance).
2. Superconductors and Superfluids
Near absolute zero, certain materials exhibit superconductivity, a state where electrical resistance drops to zero. This property has revolutionized technologies such as MRI machines, maglev trains, and particle accelerators.
Similarly, helium-4 becomes a superfluid at very low temperatures, exhibiting strange properties like flowing without friction and climbing up container walls against gravity.
3. Quantum Computing
Cooling systems near absolute zero play a critical role in quantum computing. Qubits, the fundamental units of quantum computers, rely on maintaining coherent quantum states. The ultracold environment minimizes thermal noise, enabling more stable and efficient quantum operations.
Astrophysical Context
In the vastness of space, temperatures can plummet to near absolute zero. The cosmic microwave background radiation, the afterglow of the Big Bang, measures just 2.7 Kelvin above absolute zero. This background temperature permeates the universe, setting a natural low-temperature benchmark.
Additionally, in regions like interstellar space, temperatures drop to a few Kelvin, creating environments where exotic molecules and quantum phenomena thrive. Understanding absolute zero helps scientists model and predict behaviors in these extreme cosmic conditions.
Philosophical and Practical Implications
The concept of absolute zero challenges our understanding of physical limits. It represents a boundary condition where classical physics gives way to quantum mechanics, forcing a rethinking of foundational principles. Absolute zero also symbolizes humanity’s relentless pursuit of knowledge, pushing the boundaries of what’s experimentally achievable.
From a practical perspective, research into extreme low temperatures has driven technological innovation. Cooling technologies developed in this field have applications ranging from improving semiconductor performance to developing better refrigeration systems.
Challenges and Future Directions
Despite immense progress, scientists continue to explore new methods to approach absolute zero. The development of ultra-precise temperature control systems and advancements in quantum mechanics promise further breakthroughs. Future research might reveal even more exotic states of matter or deepen our understanding of the universe’s fundamental laws.
In summary, absolute zero is not just a temperature limit but a gateway to profound scientific insights. Its pursuit has revolutionized technology and deepened our comprehension of the universe’s behavior at its most fundamental level. While we may never reach absolute zero, the journey toward it continues to be a cornerstone of scientific exploration, pushing the limits of what we can achieve and understand.